Effect of prone positioning ventilation on pulmonary function in neonates during venous-arterial extracorporeal membrane oxygenation
Highlight box
Key findings
• Prone positioning ventilation (PPV) during extracorporeal membrane oxygenation (ECMO) in newborns improves respiratory compliance and reduces airway resistance, possibly contributing to lung recovery.
What is known, and what is new?
• ECMO is an effective intervention for managing refractory respiratory and circulatory failure. PPV is a commonly used technique in critically ill infants, designed to improve thoracic pressure gradients, re-expand dorsal lung segments.
• The combination of ECMO and PPV may better improves lung function in neonatal patients.
What is the implication, and what should change now?
• PPV combined with ECMO can better improve the lung function of neonatal patients, and provide more references for clinicians to improve the respiratory function of neonatal patients under ECMO.
Introduction
Extracorporeal membrane oxygenation (ECMO) was first introduced into clinical practice in the 1960s and has proven to be an effective intervention for managing refractory respiratory and circulatory failure (1-3). Since the establishment of the International Extracorporeal Life Support Organization in 1989, by 2016, a cumulative total of 38,859 neonates had received ECMO treatment, with an overall survival rate of approximately 66% (4). Indications for neonatal ECMO typically include congenital diaphragmatic hernia, meconium aspiration syndrome, persistent pulmonary hypertension, respiratory distress syndrome, sepsis, and severe pneumonia (5). The use of ECMO technology has significantly decreased mortality rates associated with neonatal pulmonary hypertension and respiratory failure (6,7). Mugford and colleagues have posited that ECMO can notably improve survival rates in infants with reversible respiratory failure (8). Moreover, case reports from 1987 to 2006 demonstrate that as ECMO technology has advanced, the survival rates for neonates with respiratory failure post-ECMO intervention have increased from 67% to 91.1% (9).
Prone positioning ventilation (PPV) is a commonly used technique in critically ill infants, designed to improve thoracic pressure gradients, re-expand dorsal lung segments, and increase oxygenation in approximately 70–80% of patients suffering from acute respiratory distress syndrome (ARDS) by elevating the mean oxygenation index [OI = mean airway pressure × fraction of inspired oxygen (FiO2) ×100/arterial oxygen partial pressure (PaO2)] (10-13). Theoretically, combining ECMO with PPV may offer enhanced lung protection, observable through metrics such as PaO2/FiO2, OI, respiratory system compliance (Crs), airway resistance (RAW), among others. While prior studies have explored the synergistic potential of these two interventions, limited research specifically addresses the combined use of PPV and ECMO in neonatal patients (14,15). This study retrospectively analyzes clinical data from neonates who were provided ECMO support at our hospital from June 2021 to June 2023, aiming to evaluate the effectiveness and safety of integrating PPV with ECMO in the treatment regimen for these patients. We present this article in accordance with the STROBE reporting checklist (available at https://tp.amegroups.com/article/view/10.21037/tp-23-485/rc).
Methods
The study was conducted in accordance with the Declaration of Helsinki (as revised in 2013). The study was approved by the ethics board of Fujian Children’s Hospital (No. 2023ETKLRK10020) and informed consent was obtained from the legal guardians.
A retrospective study was conducted to collect clinical data from neonates diagnosed with severe ARDS who received ECMO support in our hospital from June 2021 to June 2023. Due to the evolution of clinical expertise and technology, patients with VA-ECMO were initially treated with supine positioning ventilation (SPV) from June 2021 to March 2022. Subsequently, from March 2022 to June 2023, these patients were transitioned to PPV. The primary aim of this study was to evaluate the effect of PPV in combination with ECMO on the restoration of neonatal lung function. Detailed information about the use of PPV was provided to the patient’s families, their knowing-agreeing was obtained.
Inclusion and exclusion criteria
Inclusion criteria: neonates who satisfied the Montreux Consensus Criteria and underwent ECMO therapy were included. Exclusion criteria: neonates who received ECMO support for less than 72 hours due to various reasons were excluded from the study. Utilizing our medical record system, we identified 17 cases that met the inclusion criteria. These patients were then divided based on their received treatment modality into two groups: the ECMO-PPV group (n=8) and the ECMO-SPV group (n=9).
Criteria for ECMO initiation
Neonates presenting with severe respiratory and/or cardiac failure, exhibiting a high risk of mortality, and possessing a potentially reversible condition are candidates for ECMO. They must also meet at least one of the following conditions: (I) inadequate tissue oxygenation despite maximal therapeutic efforts, indicated by rising lactate levels, worsening metabolic acidosis, or signs of end-organ dysfunction; (II) severe hypoxic respiratory failure characterized by acute decompensation (PaO2 <40 mmHg); (III) persistently elevated OI without improvement; (IV) severe pulmonary hypertension accompanied by evidence of right ventricular dysfunction and/or left ventricular failure (16).
ECMO treatment details
- Equipment: the ECMO system consisted of a centrifugal pump (MAQUET, RF-32, Hirrlingen, Germany), a membrane oxygenator (MEDOS, 800LT, Stolberg, Germany), an air-oxygen blender, a heparin-coated circuit, and a heater unit.
- Treatment mode: venous-arterial (VA) ECMO was utilized.
- On-machine process: indications for ECMO were thoroughly assessed. Suspended erythrocytes and plasma were prepared in the tubing system. Heparin was administered at 100 U/kg as an anticoagulant prior to cannulation. An 8–10 Fr arterial cannula (Medtronic, CB96825-008/010, Minneapolis, USA) was placed into the right common carotid artery, and a 10–12 Fr venous cannula (Medtronic, CB96835-010/012) was placed into the right internal jugular vein. The circuit was connected, and the flow rate was gradually increased to achieve full support (100–150 mL/kg/min). Continuous monitoring of the machine’s operation and the patient’s vital signs was conducted, adjusting the flow rates according to the individual needs of the neonates.
Ventilator support prior to ECMO
The ventilator was programmed to pressure assist/control mode. Tidal volume (Vt) was maintained at 6–8 mL/kg. Peak inspiratory pressure (PIP) ranged from 20–25 cmH2O, while positive end-expiratory pressure (PEEP) was set between 5–10 cmH2O. The respiratory rate (RR) was adjusted to 30–40 breaths per minute, and the FiO2 was set to 80–100%. The PEEP settings were tailored primarily based on the patient’s pulmonary status and arterial blood gas analysis results.
Ventilator support during ECMO
The ventilator was configured to pressure assist/control mode. Vt was maintained at 4–6 mL/kg to minimize lung volume injury. PIP was adjusted to 15–20 cmH2O, and PEEP was set between 8–10 cmH2O. RR was established at 8–10 breaths per minute, and the FiO2 was modulated to 30–50%. The targeted goals for oxygenation were to maintain an oxygen saturation above 90% or a PaO2 above 60 mmHg.
ECMO management
- At the initiation of ECMO, the centrifugal pump speed was regulated to 3,000–3,500 rpm (Rotaflow, MAQUET). The flow rate was sustained at 100–150 mL/kg/min. Typically, ECMO was started with a sweep gas set at 100% FiO2. Sweep gas flow rates were aligned with blood flow and fine-tuned to maintain PaCO2 within 40–45 mmHg and venous oxygen saturation (SvO2) between 65–80%. In cases of hyperoxia, evidenced by PaO2 exceeding 100 mmHg, the sweep gas FiO2 was decreased appropriately. Adjustments were made in response to arterial blood gas analyses.
- Heparin was administered continuously to maintain an activated partial thromboplastin time (APTT) between 60–80 seconds. Hemoglobin (Hb) levels were targeted at approximately 120–140 g/L.
- Sufentanil and midazolam were administered for adequate sedation, aiming for a Richmond Agitation-Sedation Score (RASS) between −3 and −4. (IV) Invasive blood pressure was monitored via a radial artery catheter, and the mean arterial pressure was maintained between 40–65 mmHg, prior to gradually reducing vasoactive medications (16).
Prone position ventilation strategy
Initially, the patient was placed in a supine position to clear respiratory tract secretions, after which appropriate analgesia and sedation were administered. Subsequently, a team of four medical staff carefully turned the patient into the prone position. Soft padding was placed under pressure-sensitive areas such as the forehead, shoulders, abdomen, and knees to prevent pressure ulcers. Medical staff vigilantly monitored ECMO function to ensure stable pump flow before and after the patient’s repositioning. An hourly checklist was implemented to inspect the status of ECMO cannulas, endotracheal tube, other vascular catheters, as well as gastric and urinary catheters, and chest or abdominal drains, with findings documented in the patient’s record. This checklist was also critical during nursing shift changes to ensure the security of all essential lines and tubes. Each session of PPV lasted 14 to 18 hours per day.
Data collection
We collected demographic and clinical data from patients in both groups, including gender, gestational age, age at ECMO initiation, weight, primary diagnosis, survival rate, duration of ECMO and mechanical ventilation, complications, among other variables. Comparative analyses of PaO2/FiO2, OI, Crs, RAW were conducted between the two groups before and at 1, 2, and 3 days following treatment initiation. Crs and RAW were calculated using the formulas: Crs = Vt/(plateau pressure − PEEP) and RAW = (PIP − plateau pressure)/flow, respectively. Additionally, we monitored changes in these parameters within the ECMO-PPV group before and 2 hours after the initiation of PPV.
Statistical analysis
Data analysis was conducted using SPSS version 26.0. Quantitative data were presented as mean ± standard deviation or median (interquartile range). Inter-group comparisons were performed using the two-sample t-test or non-parametric rank sum test, depending on the normality of the data. Categorical data were expressed as frequencies and percentages, with group comparisons conducted using the Chi-squared or Fisher’s exact test. A P value less than 0.05 was considered statistically significant.
Results
Two patients were excluded due to incomplete data, resulting in a total of 17 patients included in the study. Patients were divided into two groups based on the administration of PPV during their ECMO course. There were no significant differences observed between the groups regarding survival rate, mechanical ventilation duration, or complications (Table 1).
Table 1
Item | ECMO-PPV group (n=8) | ECMO-SPV group (n=9) | P |
---|---|---|---|
Male/female (n) | 4/4 | 8/1 | 0.07 |
Gestational age (weeks) | 38.07 [3.93] | 38.29 [2.71] | 0.32 |
Age at ECMO (days) | 2 [1] | 2 [2] | 0.27 |
Weight (kg) | 3.25 [0.67] | 3.15 [0.9] | 0.32 |
Pulmonary hypertension (n) | 3 | 5 | – |
Meconium aspiration (n) | 2 | 0 | – |
Lung dysplasia (n) | 2 | 2 | – |
Severe pneumonia (n) | 1 | 2 | – |
Survival rate (%) | 63 | 78 | 0.49 |
ECMO time (hours) | 78 [24.25] | 86 [19] | 0.03 |
Ventilation time (hours) | 292.5 [160.5] | 264 [100] | 0.37 |
Complications (n) | 2 | 3 | – |
VIS | 55.25±5.39 | 56.22±7.03 | 0.88 |
OI | 48.25±15.32 | 65.56±42.4 | 0.60 |
PaO2/FiO2 (mmHg) | 66.6±39.18 | 51.9±23.14 | 0.73 |
Crs (mL/cmH2O/kg) | 1.13±0.48 | 1.23±0.50 | 0.64 |
RAW (cmH2O/L/s) | 172.18±7.82 | 181.16±8.26 | 0.06 |
Data were presented as mean ± standard deviation or median [interquartile range] if not otherwise specified. ECMO, extracorporeal membrane oxygenation; PPV, prone positioning ventilation; SPV, supine positioning ventilation; VIS, vasoactive inotrope score; OI, oxygenation index; PaO2, arterial oxygen partial pressure; FiO2, fraction of inspired oxygen; Crs, respiratory compliance; RAW, resistance of airway.
Prior to the commencement of ECMO, there were no significant disparities in PaO2/FiO2, OI, Crs, or RAW between the groups (Table 1). As the ECMO treatment continued, both groups showed gradual improvements in PaO2/FiO2 and Crs, along with reductions in OI and RAW. Notably, on the third day of treatment, the ECMO-PPV group exhibited a statistically significant increase in Crs and decrease in RAW compared to the ECMO-SPV group (Crs P=0.04, RAW P=0.03) (Table 2).
Table 2
Variables | Subgroups | OI | PaO2/FiO2 (mmHg) | Crs (mL/cmH2O/kg) | RAW (cmH2O/L/s) |
---|---|---|---|---|---|
ECMO-SPV group | Before ECMO | 65.56±42.4 | 51.9±23.14 | 1.23±1.10 | 181.16±8.26 |
1 day after ECMO | 4.16±0.72 | 224.5±36.17 | 1.37±0.72 | 172.25±11.58 | |
2 days after ECMO | 4.09±0.62 | 235.49±37.13 | 1.52±0.74 | 161.47±12.25 | |
3 days after ECMO | 4.03±0.65 | 231.96±32.76 | 1.56±0.90 | 157.23±9.01 | |
ECMO-PPV group | Before ECMO | 48.25±15.32 | 66.6±39.18 | 1.13±0.48 | 172.18±7.82 |
1 day after ECMO | 4.04±0.55 | 233.1±33.36 | 1.33±0.32 | 166.84±8.50 | |
2 days after ECMO | 3.91±0.54 | 241.3±40.12 | 1.66±0.37 | 151. 48±9.26 | |
3 days after ECMO | 3.88±0.42 | 244.7±34.44 | 1.73±0.55# (P=0.04) | 132.33±8.18# (P=0.03) |
Data were presented as mean ± standard deviation. #, means compared with the ECMO-SPV group in the same period, the difference was statistically significant. OI, oxygenation index; PaO2, arterial oxygen partial pressure; FiO2, fraction of inspired oxygen; Crs, respiratory compliance; RAW, resistance of airway; ECMO, extracorporeal membrane oxygenation; PPV, prone positioning ventilation; SPV, supine positioning ventilation.
In the ECMO-PPV group, after 2 hours of PPV, Crs showed variable improvements, and RAW decreased correspondingly. These differences reached statistical significance by the third day (Crs P=0.03, RAW P=0.03). Before the next PPV session, parameters including PaO2/FiO2, OI, Crs, and RAW exhibited some variability, indicating temporary persistence of PPV effects. Prolonged PPV sessions appeared to augment recovery of pulmonary function in these neonates (Figure 1).
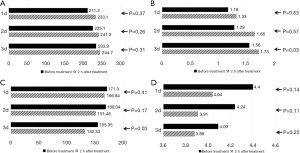
In terms of complications, three patients in the ECMO-PPV group experienced bleeding: two had ventricular hemorrhages and one had thoracic hemorrhage. In the ECMO-SPV group, two patients developed complications: one had ventricular hemorrhage and one had atrial thrombus. Minor first-degree pressure ulcers were observed in four neonates from the ECMO-PPV group and three from the ECMO-SPV group, requiring no specialized treatment. There were no reported incidents of slippage of arteriovenous cannulas, gastric tubes, catheters, or other drainage tubes, nor were there any reports of ECMO cannula site loosening, significant dislocation, or other severe adverse events.
Discussion
PPV was adopted to improve oxygenation in patients suffering from anoxic acute respiratory failure while under mechanical ventilation. Studies suggest that PPV is a critical intervention for severe hypoxemia (17). Key mechanisms driving enhanced oxygenation through PPV include redistribution of lung density, re-expansion of dorsal lung segments, increased chest wall compliance, reduced alveolar shunting, improved ventilation-perfusion ratio, better oxygenation and carbon dioxide elimination, and decreased ventilator-induced lung injury (18,19). Additionally, PPV enhances lung protection. In ARDS scenarios, some alveoli may cyclically open and close due to pressure changes from mechanical ventilation, intensifying shear forces and potentially worsening alveolar damage. PPV positively affects the gravitational pressure gradient and the distribution of edematous fluids, which facilitates consistent ventilation across the lung and reduces inter-alveolar shear forces. The prone position further assists in the drainage of pulmonary secretions, especially in lower and dorsal lung segments, thus optimizing ventilation (20). Neonates with acute respiratory failure often present with underlying issues such as pulmonary hypertension, meconium aspiration, lung dysplasia, or severe pneumonia, all originating from increased pulmonary vascular resistance and a mismatched ventilation-perfusion ratio. Additionally, their inherently fragile lung function predisposes them to atelectasis (21). Brüssel and colleagues noted that in patients with acute respiratory failure, PPV reduced atelectasis and improved oxygenation due to the shift of ventilation from ventral to dorsal regions, thereby enhancing dorsal ventilation-perfusion matching (22).
In our study, no significant differences were observed between groups regarding survival rates. While not reaching statistical significance, the ECMO-PPV group exhibited a prolonged duration of mechanical ventilation compared to the ECMO-SPV group. This observation may be attributed to the limited sample size in this study, and the potential data bias from individual patients could influence the results. Future endeavors will involve expanding the sample size to mitigate the impact of data bias. Following ECMO initiation, both groups showed improvements in PaO2/FiO2 ratios and OI, with the ECMO-PPV group demonstrating more notable increases in PaO2/FiO2. Notably, 2 hours after PPV initiation, there was an increase in the PaO2/FiO2 ratio and a decrease in OI compared to pre-PPV values, highlighting the potential of PPV to improve oxygenation in neonatal ECMO patients. While no significant differences in Crs were observed between the ECMO-SPV and ECMO-PPV groups on the first and second days following ECMO initiation, an increase in Crs was observed in the ECMO-PPV group 2 hours after PPV initiation compared to pre-PPV values. This increase became significantly pronounced by the third day of treatment, indicating improved airway compliance in the ECMO-PPV group. Similarly, RAW decreased in both groups, with a more significant reduction in the ECMO-PPV group, reaching statistical significance by day 3. However, these enhancements in Crs and RAW were not sustained after returning to a supine position before the next PPV session, implying that more frequent and potentially longer PPV sessions might benefit neonates by maintaining these improvements.
Major complications associated with PPV include the inadvertent dislocation or removal of central venous catheters, endotracheal tubes, chest drains, or ECMO cannulas. The ECMO-PPV group, due to the multitude of invasive medical devices involved, faced an increased risk of serious complications, particularly when in the prone position, which can complicate nursing care and increase the potential for oversight. Therefore, the creation of a detailed and reliable nursing checklist, along with meticulous record-keeping and regular shift handovers, is crucial. Previous studies indicate that PPV concurrent with ECMO is both safe and feasible (23,24). Marco Giani’s multicenter adult study observed that PPV during ECMO was linked to decreased in-hospital mortality and enhanced oxygenation (25). In our study, minor first-degree pressure ulcers were observed in four neonates from the ECMO-PPV group and three from the ECMO-SPV group, requiring no specialized interventions. This suggests that enhancing nursing education and implementing comprehensive operational checklists can maximize the benefits of PPV for neonates on ECMO, outweighing the risks of potential complications.
Limitations
This study was conducted at a single center with a limited sample size. Due to the relatively few neonates with ARDS receiving ECMO, there remains uncertainty about the optimal indications, treatment duration, termination criteria, and prognostic impact of PPV during ECMO. The retrospective nature of the analysis may have led to underreporting of potential complications. We considered comparing supine and prone ventilation within the same patient cohort, but the limited sample size might result in significant variability in outcomes. Despite these limitations, we believe the findings hold some clinical relevance. However, the interpretation of oxygenation outcomes must account for the influence of both the ventilator and ECMO, and thus our conclusions should serve as empirical guidance. Furthermore, our results are specific to neonates on ECMO and may not be generalizable to other patient demographics. Therefore, to substantiate these findings, larger, multicenter, prospective, randomized, double-blind, long-term studies are necessary.
Conclusions
Implementing PPV in neonates undergoing ECMO may improve respiratory compliance and decrease RAW, potentially aiding in the recovery of lung function. Moreover, our study suggests that PPV serves as a viable alternative intervention for neonates receiving ECMO support.
Acknowledgments
We highly acknowledge the following researchers’ contributions: Xiu-Xuan Ruan, Li-Wen Wang, Qi-Liang Zhang, Wang-Sheng Dai, Ze-Wei Lin, Zeng-Chun Wang, and Ling-Shan Yu.
Funding: None.
Footnote
Reporting Checklist: The authors have completed the STROBE reporting checklist. Available at https://tp.amegroups.com/article/view/10.21037/tp-23-485/rc
Data Sharing Statement: Available at https://tp.amegroups.com/article/view/10.21037/tp-23-485/dss
Peer Review File: Available at https://tp.amegroups.com/article/view/10.21037/tp-23-485/prf
Conflicts of Interest: All authors have completed the ICMJE uniform disclosure form (available at https://tp.amegroups.com/article/view/10.21037/tp-23-485/coif). The authors have no conflicts of interest to declare.
Ethical Statement: The authors are accountable for all aspects of the work in ensuring that questions related to the accuracy or integrity of any part of the work are appropriately investigated and resolved. The study was conducted in accordance with the Declaration of Helsinki (as revised in 2013). The study was approved by the ethics board of Fujian Children’s Hospital (No. 2023ETKLRK10020) and informed consent was obtained from the legal guardians.
Open Access Statement: This is an Open Access article distributed in accordance with the Creative Commons Attribution-NonCommercial-NoDerivs 4.0 International License (CC BY-NC-ND 4.0), which permits the non-commercial replication and distribution of the article with the strict proviso that no changes or edits are made and the original work is properly cited (including links to both the formal publication through the relevant DOI and the license). See: https://creativecommons.org/licenses/by-nc-nd/4.0/.
References
- Abrams D, Bacchetta M, Brodie D. When the momentum has gone: what will be the role of extracorporeal lung support in the future? Curr Opin Crit Care 2018;24:23-8. [Crossref] [PubMed]
- Abrams D, Brodie D. Extracorporeal Membrane Oxygenation for Adult Respiratory Failure: 2017 Update. Chest 2017;152:639-49. [Crossref] [PubMed]
- Stroud MH, Okhuysen-Cawley R, Jaquiss R, et al. Successful use of extracorporeal membrane oxygenation in severe necrotizing pneumonia caused by Staphylococcus aureus. Pediatr Crit Care Med 2007;8:282-7. [Crossref] [PubMed]
- Thiagarajan RR, Barbaro RP, Rycus PT, et al. Extracorporeal Life Support Organization Registry International Report 2016. ASAIO J 2017;63:60-7. [Crossref] [PubMed]
- International ECLS Registry Report. Extracorporeal Life Support Organization; Ann Arbor, MI. January 2017.
- Kim F, Bernbaum J, Connelly J, et al. Survival and Developmental Outcomes of Neonates Treated with Extracorporeal Membrane Oxygenation: A 10-Year Single-Center Experience. J Pediatr 2021;229:134-140.e3. [Crossref] [PubMed]
- Bennett CC, Johnson A, Field DJ, et al. UK collaborative randomised trial of neonatal extracorporeal membrane oxygenation: follow-up to age 4 years. Lancet 2001;357:1094-6. [Crossref] [PubMed]
- Mugford M, Elbourne D, Field D. Extracorporeal membrane oxygenation for severe respiratory failure in newborn infants. Cochrane Database Syst Rev 2008;CD001340. [Crossref] [PubMed]
- Schaible T, Hermle D, Loersch F, et al. A 20-year experience on neonatal extracorporeal membrane oxygenation in a referral center. Intensive Care Med 2010;36:1229-34. [Crossref] [PubMed]
- Kipping V, Weber-Carstens S, Lojewski C, et al. Prone position during ECMO is safe and improves oxygenation. Int J Artif Organs 2013;36:821-32. [Crossref] [PubMed]
- Antunes LC, Rugolo LM, Crocci AJ. Effect of preterm infant position on weaning from mechanical ventilation. J Pediatr (Rio J) 2003;79:239-44. [Crossref] [PubMed]
- Oishi Y, Ohta H, Hirose T, et al. Combined effects of body position and sleep status on the cardiorespiratory stability of near-term infants. Sci Rep 2018;8:8845. [Crossref] [PubMed]
- Fioretto JR, Klefens SO, Pires RF, et al. Comparison between conventional protective mechanical ventilation and high-frequency oscillatory ventilation associated with the prone position. Rev Bras Ter Intensiva 2017;29:427-35. [Crossref] [PubMed]
- Miller AG, Bartle RM, Rehder KJ. High-Frequency Jet Ventilation in Neonatal and Pediatric Subjects: A Narrative Review. Respir Care 2021;66:845-56. [Crossref] [PubMed]
- Lu H, Zhang P, Liu X, et al. Effect of prone position ventilation on right heart function in patients with acute respiratory distress syndrome. Clin Respir J 2021;15:1229-38. [Crossref] [PubMed]
- Wild KT, Rintoul N, Kattan J, et al. Extracorporeal Life Support Organization (ELSO): Guidelines for Neonatal Respiratory Failure. ASAIO J 2020;66:463-70. [Crossref] [PubMed]
- Lupton-Smith A, Argent A, Rimensberger P, et al. Prone Positioning Improves Ventilation Homogeneity in Children With Acute Respiratory Distress Syndrome. Pediatr Crit Care Med 2017;18:e229-34. [Crossref] [PubMed]
- Guerin C, Baboi L, Richard JC. Mechanisms of the effects of prone positioning in acute respiratory distress syndrome. Intensive Care Med 2014;40:1634-42. [Crossref] [PubMed]
- Gattinoni L, Busana M, Giosa L, et al. Prone Positioning in Acute Respiratory Distress Syndrome. Semin Respir Crit Care Med 2019;40:94-100. [Crossref] [PubMed]
- Galiatsou E, Kostanti E, Svarna E, et al. Prone position augments recruitment and prevents alveolar overinflation in acute lung injury. Am J Respir Crit Care Med 2006;174:187-97. [Crossref] [PubMed]
- Albert RK, Hubmayr RD. The prone position eliminates compression of the lungs by the heart. Am J Respir Crit Care Med 2000;161:1660-5. [Crossref] [PubMed]
- Brüssel T, Hachenberg T, Roos N, et al. Mechanical ventilation in the prone position for acute respiratory failure after cardiac surgery. J Cardiothorac Vasc Anesth 1993;7:541-6. [Crossref] [PubMed]
- Shi J, Wang C, Cui Y, et al. Extracorporeal membrane oxygenation with prone position ventilation successfully rescues infantile pertussis: a case report and literature review. BMC Pediatr 2018;18:377. [Crossref] [PubMed]
- Marinelli L, Mori L, Avanti C, et al. Meralgia Paraesthetica after Prone Position Ventilation in a Patient with COVID-19. Eur J Case Rep Intern Med 2020;7:002039. [Crossref] [PubMed]
- Giani M, Martucci G, Madotto F, et al. Prone Positioning during Venovenous Extracorporeal Membrane Oxygenation in Acute Respiratory Distress Syndrome. Ann Am Thorac Soc 2021;18:495-501. [Crossref] [PubMed]